
Electrification won’t break the grid, it will make it smarter.
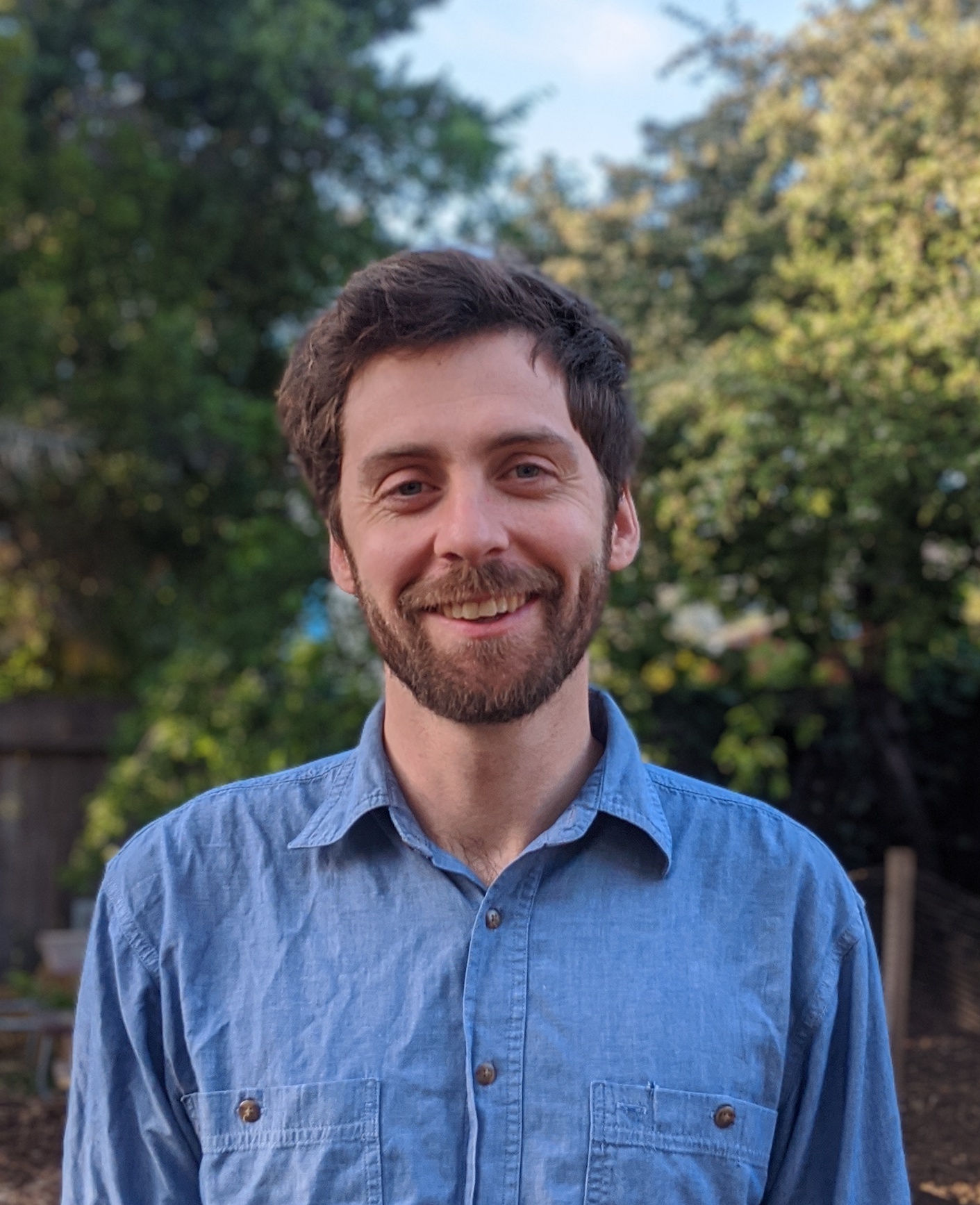
Sam Calisch
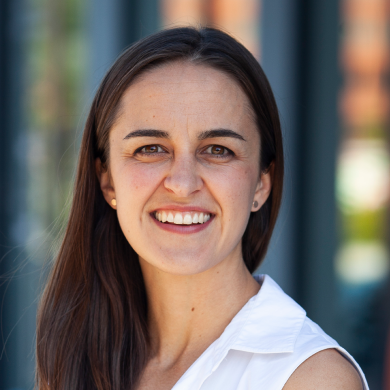
Cora Wyent
Director, Research
-
To meet our climate goals, we’ll need to deliver two to three times as much electricity as today. Fortunately, we’ve scaled the grid faster than this before and can do it again [1].
-
Smart grid technologies can allow more electricity to be delivered by the same infrastructure, reducing the amount of new grid build-out and increasing resilience.
-
Smart electric panels can enable whole home electrification, including EVs and heat pumps, largely without upgrading upstream infrastructure like wires or transformers, cutting the cost and time required for electrification.
To meet our climate goals, we must electrify nearly everything in our economy. That will require delivering about two to three times more electricity than we do today [2]. A share of this will come from clean solar energy generated right on our rooftops [3], but our grid will also need to grow significantly to deliver the remaining electricity demand. Skeptics of electrification say the grid won’t be able to handle this [4], but history says otherwise. In just one decade, between 1950 and 1960, the amount of electricity delivered more than doubled in the U.S, and by 1970 it had more than doubled again [5], largely in response to the proliferation of uses for electricity beyond lighting [6]. As American households now similarly spur increased electricity demand to power electric vehicles and heat pumps [7], we can be confident that we can deliver, because we’ve done it before.
Text with Image (The Grid Circuit Breaker)
In order to grow the grid as efficiently and effectively as possible, we can leverage a host of technologies that make it smarter, more responsive and capable of delivering more electricity [8]. The cost of infrastructure – like wires and transformers – is driven by assumptions about worst case demand: the grid is typically designed to operate even if all consumers were to turn on nearly all of their devices at the same time. Because these events are so exceedingly rare, we aren’t using these components to their full potential. A smarter grid would use the inherent flexibility of electricity demand [9] to avoid these situations altogether, allowing more electricity to be delivered by the same infrastructure.
While this may seem far-away and complex, there are significant opportunities hiding right in our basements and utility closets, embodied in the humble electrical panel [10]. These pieces of infrastructure are responsible for safely regulating the flow of electricity between the grid and loads in a building.
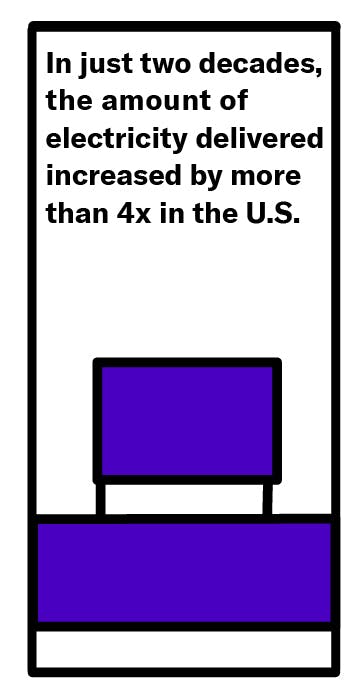
As homes electrify, adopting electric vehicles, heat pumps and electric stoves in place of fossil fuel machines, they may demand more electricity than the panel was originally designed for. Like other grid infrastructure, panels are sized for the peak electricity load: roughly the amount of electricity required if your electric vehicles, heat pump, water heater, stove and everything else is turned on at the same time [11]. This peak load, however, is 10-20 times higher than the average load over time [12]. Thus, if we can better manage peak events, substantially more electricity can be delivered without increasing panel capacity.
Fortunately, we have “smart panels”, which are electrical panels designed to do just this, measuring the flow of electricity and allowing individual circuits to be automatically turned on and off to regulate a home’s peak electricity demand. As an example, in Figure 1 at left, we show a peak event from an actual electrified home around dinner time, using data provided by SPAN, a leading manufacturer of smart panels. The householder likely arrived home from work, plugged in their car, started a load of laundry and turned up the thermostat. As a result, the total electricity drawn from the grid exceeded 80 amps for several minutes [13], exceeding a safe limit for a 100 amp panel [14]. If not for a smart panel, this household would likely have needed a 200 amp service upgrade when electrifying. On the right, we see the same event as could be mediated by the smart panel. By shifting 34 minutes of EV charging (shown in orange) with no inconvenience to the householder, the maximum load is kept below that 80 amp threshold at all times [15]. In data from SPAN’s electrical panels covering all contiguous U.S. climate zones, over 80 percent of peak events [16] were less than 12 minutes long, and shifting the operation of just one of the water heater, dryer, EV charger, or HVAC system can mitigate 90 percent of all peak events. These data indicate that the vast majority of peak loads could be shifted without any noticeable effect to the household.
Figure 1: Left) A real-world peak event of over 80 amps around dinner time, caused by an EV charger, heat pump, water heater, and dryer all cycling on at the same time. Right) The same event, with the current peak held below 80 amps by shifting 34 minutes of EV charging (orange). Data provided by Span.IO, Inc. and analyzed by Rewiring America.
Today, approximately 50-60 million single-family homes (or approximately 60-70 percent) have electrical panels with ratings less than 200 amps [17]. If these households fully electrify (including two electric vehicle chargers, heat pump HVAC, heat pump water heating, electric range, and electric dryer), it is likely that most will need a new, larger electrical panel [18]. If this new panel is not a smart panel, a further upgrade to the incoming electrical service (that is, the wires carrying electricity from the street) may also be required. While buying a smart panel is incrementally more expensive than a conventional panel, the avoided costs of a resulting service upgrade can be hefty [19]. Particularly for households receiving electrical service through underground wires, the avoided costs are likely to pay for the smart panel upgrade many times over.
To encourage electrification, policymakers should provide incentives to homeowners to reduce the costs of panel upgrades, especially for low- and moderate-income households. For example, the climate provisions of the 2021 budget reconciliation bill passed by the House of Representatives [20] included direct rebates of up to $3,000 for this. The Senate should move quickly to pass this legislation with these measures intact. Utilities can also play a role, providing information on smart panels and incentives to defray the upfront cost premium when a customer applies for a service upgrade [21]. Further, the National Fire Protection Association’s Committee on the National Electrical Code should modernize regulations on electrical panel sizing to clarify that smart panels allow extra load to be added to an existing electrical service. Finally, in cases where a service upgrade is unavoidable, utility regulators should reallocate subsidies for natural gas connections [22] to electrical service upgrades.
Our grid today is built for the worst-case scenario: the short, rare instances where households are using all of their machines at once. This is an inefficient use of electric infrastructure, and makes scaling our electric grid to support an increasingly electrified economy harder than it needs to be. Smart grid technologies like smart panels can maintain the necessary reliability and resiliency of the grid, while allowing us to scale our infrastructure more efficiently and cost-effectively.
Acknowledgement
The authors would like to thank Joel Rosenberg, Steve Pantano, Ari Matusiak and Leah Stokes of Rewiring America for helping bring this piece together. Additionally, thanks to Kelsey Wallace, Chad Conway, Brad Davids, Arch Rao, Julia Sachs and Jan Overgoor of SPAN for access to data from a fleet of smart panels and helpful discussions of the role of smart panels in electrification.
Notes
[1] Grid expansion and resilience is a huge topic – in this article we focus on technologies near the point of use, namely smart panels. Consider this to be part one of a series on the larger topic.
[2] In The Rewiring America Handbook (Saul Griffith, Sam Calisch and Laura Fraser, July 2020) and Electrify (Saul Griffith, MIT Press, 2021), the authors show how the U.S. economy can be run on roughly 45 Quads of energy per year. Over the 8,760 hours in the year, this amount of energy is equivalent to an average power of 1500GW. Arguing conservatively, we ignore the biofuels and other non-electric energy sources likely to be responsible for a portion of this total, and assume all of this energy is supplied by electricity. In contrast, according to the EIA, today we deliver approximately 4.1 million GWh over the 8,760 hours of the year. This is equivalent to an average power of approximately 470 GW. Thus, in this nearly-completely electrified future scenario, we need to supply about 3.2 times as much electricity as we do today.
The IPCC also estimates the increase in electricity delivered, assuming the use of biofuels, carbon capture and sequestration, hydrogen, and significant behavior change for demand side mitigation. To achieve a likely 2°C temperature rise, approximately 200 to 225 EJ of electricity per year are required globally (See AR6 WGIII Figure 3.23). This represents an increase of approximately 2.2 to 2.5 times over today’s levels.
In the IEA Net Zero scenario (see Net Zero by 2050 - A Roadmap for the Global Energy Sector Figure 3.9), the electricity demand is expected to double by 2050 in “advanced economies” like the U.S.
These estimates are based on a top-down energy transition model based on climate goals. In contrast, other studies use a bottom-up technology adoption model based on current trends. These studies generally find the increase to the amount of delivered electricity to be lower. For example, in NREL’s Electrification Futures Study, the “High” adoption scenario projects an increase in delivered electricity of roughly 160%. This scenario is based on “a combination of technology breakthroughs, policy support, and underlying societal and behavioral shifts that yield an electrification transition.” The rate of adoption is “impacted by a wide range of factors including economic trade-offs, consumer preference, and policies”.
[3] According to a 2016 NREL study, our collective rooftops represent about 1,118 GW of potential solar capacity. After subtracting the roughly 27GW of small scale solar currently installed (see EIA Table 4.3. Existing capacity by energy source), the difference is remarkably close to the additional capacity needed, even at the upper estimates of increased demand. However, after taking into account the economic feasibility of each installation, and the need to supply power to match demand, the amount likely to be deployed is likely less.
[4] Much of this skepticism is not based in fact, but there are legitimate concerns about ensuring adequate investment in the grid. In places where adoption of electrification has taken off and investments have not kept pace, there are signs of strain. For a good narrative description of such a scenario, see Old Power Gear Is Slowing Use of Clean Energy and Electric Cars, Ivan Penn, The New York Times. For quantitative analysis, see Can Distribution Grid Infrastructure Accommodate Residential Electrification and Electric Vehicle Adoption in Northern California?, Anna Brockway, Duncan Callaway, and Salma Elmallah, June 2022.
[5] According to the EIA’s Table 7.2a Electricity Net Generation: Total (All Sectors), in 1950, 334 GWh were delivered. By 1960, 759 GWh were delivered, a 227% increase. By 1970, 1,535 GWh were delivered, a 202 percent increase over 1960 and a 460 percent increase over 1950!
[6] Major national campaigns were used in this time to spread public awareness, including the Live Better Electrically: The Gold Medallion Electric Home Campaign by General Electric and Westinghouse. This campaign extolled the virtues of electric appliances and featured then-actor Ronald Reagan as the main spokesperson.
[7] See Argonne National Lab’s Light Duty Electric Drive Vehicles Monthly Sales Updates and the Air Conditioning, Heating, and Refrigeration Institute’s Central Air Conditioners and Air-Source Heat Pumps Sales Data.
[8] To date, these technologies have been referred to as the “smart grid”, where information about grid conditions is passed alongside the electricity in order to increase resilience and efficiency. See Grid Modernization and the Smart Grid | Department of Energy and Demand Response | Department of Energy.
[9] See FERC’s National Action Plan on Demand Response for examples.
[10] The electrical panel (also known as breaker box, panelboard, distribution board, switch box, and probably more) is responsible for safely delivering electricity from the utility’s power lines to your home’s outlets and appliances. Electricity first travels from the power lines on the street through either underground or above-ground service wires. It passes through a second set of service entrance wires, through your meter, and then arrives at your electrical panel. The electrical panel splits the electricity into different circuits for the locations and appliances in your home. The utility owns the service wires and the meter, and the customer owns everything else: the service entrance wires, the electrical panel, and the wires that distribute electricity throughout the home.
[11] When installing a new circuit for such an appliance, an electrician sizes an electrical panel by calculating a given home’s peak electricity usage, summing up how much electricity all of the appliances and loads would use if they were turned on at the same time. An example of an electrical load calculation worksheet is shown here. A few example homes are shown on Redwood Energy’s Watt Diet Calculator, and Pecan Street’s study Addressing an Electrification Roadblock: Residential Electric Panel Capacity. A typical electrified 2000-square foot home might have approximately the following loads:
- Lighting = 6 kVA
- EV charger = 10 kVA
- Electric cooktop & oven = 10 kVA
- Electric dryer = 5 kVA
- Heat pump (no back-up heat) = 5 kVA
- Heat pump water heater = 5 kVA
- Dishwasher, microwave, washing machine, garbage disposal, 2 x other appliances = 1.5 kVA each
This sums to 50 kVA total. Per the National Electric Code (NEC), to size an electrical panel, we sum up 1) 100 percent of the first 10 kVA, then 40 percent of the remaining kVA, for lighting and appliance loads, and 3) all of the heating/cooling load (whichever is greater if separate appliances are used for heating and cooling). For this home, that yields 10 + 0.4*35 + 5 = 29 kVA, which is 121 A after dividing by 240V. With traditional electrical panels and no circuit-sharing devices, this home would need a 150 A or 200 A electrical panel. Having solar panels could further increase the necessary amperage.
[12] This figure is based on data from over 1,000 homes with smart panels provided by SPAN. Peak loads are evaluated as a maximum of current measurements taken every second through the main breaker, while averages are the total energy delivered through the main breaker divided by the time in operation. There is some regional variation in this ratio, with the “Very Cold” climate region having average values near 20, while “Mixed Humid” region had values of approximately 10. See DOE Building America Climate Zone Guide for climate zone definitions.
[13] The amp (short for ampere) is the unit of electrical current, or flow of electricity. Electrical panels are measured by how many amps they can provide. Older, smaller houses may have panels that can provide 60 or 100 amps, while newer, larger houses likely have 200 amp panels.
[14] This can be confusing, but in short, 80 amps is considered a safe, conservative, continuous load limit for a 100 amp panel. The National Electric Code rules for load sizing are complex (see NFPA 70: National Electrical Code) and not designed with active load management in mind. However, conductor sizes and overcurrent protection for feeders and services are specified so continuous loads are limited to 80 percent of the overcurrent limit (See 215.3 and 230.42(A)(1) of the NEC). Further, most standard circuit breakers themselves are only designed to sustain 80 percent of their nameplate ratings for an extended period of time (i.e., 80 percent of the number of amps that will cause the breaker to trip and disconnect the circuit). See Clearing up Confusion over 80% vs. 100%-rated Circuit Breakers, Schneider Electric Blog. Even with continuous loads limited to 80 amps, startup surges will create brief spikes above this baseline. If the continuous load baseline were higher, these spikes would frequently trip the main breaker, cutting power to the house and inconveniencing the homeowner.
Finally, the house from which this data comes actually had a panel larger than 100 amps, but we use this threshold to illustrate the common case of electrification with this panel size.
[15] The majority of home electrical loads, like water heaters and HVAC equipment, operate cyclically, turning full-on for a period, and then full-off for a period, supplying the right amount of average power to keep the house comfy and the water hot. Because of this, under most conditions, such loads can be synchronized to avoid turning on at the same time without any noticeable interruption in service to the homeowner. Other loads (like electric vehicle charging) can be easily shifted within a window of time (e.g., when the car is plugged in) in order to avoid drawing too much electricity.
[16] Here “peak event” is defined as any instance where total load exceeds 80 amps.
[17] To our knowledge, there is no complete data on US-wide panel sizes. Three independent sources give us the 60 percent estimate. First, the Addressing an Electrification Roadblock: Residential Electric Panel Capacity study from Pecan Street looked at a sample of 263 Texas homes and extrapolated to the US, finding that 48 million single-family households (out of 86 million total single-family households) would likely need a panel upgrade in order to electrify. In their Texas sample, 60 percent of homes have electrical panels sized below 200A. Second, the Service Upgrades for Electrification Retrofits Study Draft Report estimates that 70 percent of California homes have at least 100A panels based on year of construction. (This overestimates the number of <200A panels because it includes those with larger panels, but underestimates because it excludes those who upgraded their panels to install air conditioning. As all new construction in CA must have 200A panels, we assume that the number with less than 200A panels is around 60 percent). Third, we can assume as a general rule of thumb that single-family homes 2500 square feet and over have at least 200A panels, and most homes that are less than 2500 square feet have less than 200A panels. According to the Residential Energy Consumption Survey, about 70 percent of US homes are under 2500 square feet in size. This is certainly a rough estimate, and better data on electrical panel sizes across the US is needed.
[18] There are additional methods that allow households to electrify while avoiding electrical panel and service upgrades in some cases. Circuit-sharing plugs allow two colocated appliances that use a lot of electricity, like an EV charger and a clothes dryer in a garage, to share a plug and gracefully avoid turning on at the same time. Two examples of companies that sell circuit-sharing devices are NeoCharge and Splitvolt. Smart breakers can also provide some of the functionality of a smart panel for individual circuits. Another option is use of a meter collar, such as this one from Siemens and ConnectDER, which installs between the existing electric meter and meter socket. In addition to avoiding upgrading the electric panel, these collars can monitor the total load and modulate the downstream circuit (such as an EV charger) to stay within the limits of the incoming service.
Alternatively, households can also select power-efficient appliances and lower amp EV chargers to keep their electrical needs under 100 amps. Several handy electricity budget calculators exist to help with this. For instance, Redwood Energy’s Watt Diet calculator can help households and electricians size electrical panels, and select power-efficient appliances in order to avoid panel and service upgrades. For example, although 50-amp circuits are commonly used for EV charging, a 20-amp circuit can provide 100 miles of charge overnight, more than enough for most people to get to and from work each day. Power-efficient appliances are coming to the market, including 120-volt heat pump water heaters, 120-volt stovetops and ranges, and 120-volt ductless mini-split heat pumps, as well as lower-amp versions of those appliances.
[19] Electrical service upgrades can be lengthy and expensive, involving swapping out the wires that connect your house to the power lines on the street with larger ones. In some cases, it may also involve some other upstream upgrades, such as swapping out transformers and high voltage power lines. Because of the need for trained technicians and shutting off electricity to the lines, service upgrades can take upwards of six months, and can cost anywhere from $2,000–$30,000, without including the electrical panel upgrade. Though the utility typically covers a portion of the costs, even the costs that the utility covers will ultimately be distributed among ratepayers. If we assume a cost of $5,000 per home for a service upgrade, the cost to upgrade electrical service on all homes in the U.S. would be roughly $250 billion.
The total project costs can be broken into three categories: 1) the cost of the electrical panel, 2) the cost of the service line replacement, and 3) other related costs, such as replacing the pole and transformer. Within each of these categories, there are costs associated with labor, materials, and permits, and all are incorporated into the following cost estimates. The cost of the electrical panel is always the customer’s responsibility, and can range from $2,000–$4,500. The cost of the service line replacement is supposed to be covered by a service extension allowance, which is in the range of $2000–$3000 and represents the average cost paid by the utility. Customers are responsible for any costs above that allowance. Any project that is not a simple above-ground line replacement (e.g. if service wires are underground) is likely to exceed the allowance, and can cost up to $16,000. Replacing the pole and the transformer is the customer’s responsibility if they are the only customer on the transformer, the utility’s responsibility if there are three or more customers on the transformer, and split otherwise. A transformer replacement can cost $6,000–$8,000, and a pole replacement can cost $9,000–$11,000. In total, the cost to upgrade the electrical service (not including the electrical panel upgrade) can range from $2,000 to upwards of $30,000. See the Service Upgrades for Electrification Retrofits Study Draft Report.
Beyond cost, the long timelines of service upgrades can be a deterrent for consumers considering electrification, and can often result in customers deciding instead to purchase new gas-powered appliances, locking in fossil fuel usage for decades.
[20] In the text passed by the House (see 117th Congress (2021-2022): Build Back Better Act), electric service panels rebates are included in the High-Efficiency Electric Home Rebate program. In the Senate finance committee text (see TITLE XII—COMMITTEE ON FINANCE), electric panels are also included in the 25C residential energy property tax credit.
[21] It is important to note that the ideal time for a customer to install a smart panel is when they are already doing a panel upgrade, since there is typically no incremental installation cost, only a minor incremental hardware cost.
[22] For example, see Revisiting California's natural gas hookup subsidies, Utility Dive.